Urchin Sperm Cell Hydrodynamics
Story about my scientific paper
Experimental data: article by U.B. Kaupp and others. The signal flow and motor response controlling chemotaxis of sea urchin sperm.
Urchins (Echinoidea) — small and spiky sea animals, phylum: echinoderms.

During my late years at the University I was engaged in modeling hydrodynamics of an urchin’s sperm. I loved this task and that’s why I decided to share it, although this topic is not in any way related to information design.
The model was built around experimental data received by German scientists and connected the internal biochemical processes with the macroscopic motion of the sperm.
Biophysics and Biochemistry
The coating of eggs laid by female urchins contains a special chemical compound. It dissolves in water and creates sort of an attractive aura around the ovum. The sperm are equipped with sensitive receptors transfering the signal to the flagellum’s engine upon the contact with the molecules of this secretion. The combination of signals from various molecules defines the pattern of the flagellum’s motions and directs the sperm to the target.
A model of this process explained how the sperm cell “understands” which direction to take basing on distinct sporadic signals from receptors.
Physics and Mathematics
The urchin sperm cells travel through sea water. The environment was modeled by the cellular automaton, since it pretty accurately describes the dynamic processes of movement in liquid.
The cellular automaton is a mathematical model in which time and space are discrete. Physical space is represented by a uniform grid and the state of cells of a cellular automaton is defined by the presence of particles in them, which move parallel to the axes of not more than one particle in each direction.

All possible states of the cells of a two-dimensional cellular automaton
The initial distribution of particles in the field of a cellular automaton is defined arbitrarily. At every step the particles move one cell at a time following the direction of motion, changing the cell’s state.

If one cell contains particles moving in opposite directions, we are talking about the collision of the cellular automaton particles. After the collision, the particles fly apart and their directions are perpendicular to the original directions. This is equivalent to the full exchange of momentum between the particles, where the laws of the conservation of energy and momentum are observed. The repulsion of particles from the border of the cellular automaton occurs in a similar rules.
An object of simplified shape with the border outlining the cell boundaries is moving in discrete “water” instead of a real sperm. The cells of the cellular automaton induce hydrodynamic pressure against the surface of the modeled sperm by repulsing from this border.

Sea urchin sperm
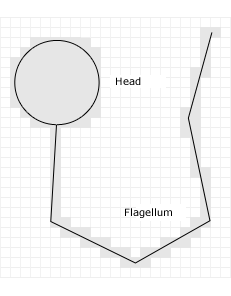
Model object of a simplified shape in the cellular automaton’s field
During the first run of the program the sperm swam tail backwards. I had to recheck all the records before I found the lost “minus” sign :-)
Result Represenatation
The program generates on average 150 images per cycle. An experimental article that I worked on suggested the way to visualize them properly. In the article, the pictures of a moving sperm were superimposed one over another.
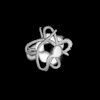

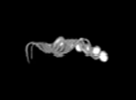
Pictures from the article: swimming around a small radius circle, turn, in-line motion.
This technique worked perfectly with the modeling pictures (animation on hover!)